jim Chatfield — June 1, 2011
The best design and the healthiest plants won’t survive in compacted, compromised soil. Before you plant, know what you’re working with. You’ll learn about soil basics in Part 1. And watch the July issue for Part 2, where you’ll learn how to improve the urban soil you encounter.
Soil that has been damaged by construction activity is the bane of every landscaper. Few landscapers have the opportunity to work with contractors to minimize soil abuse during construction. Most often, horticultural concerns take a back seat to architectonics. While some damage to the soil is inevitable during suburban construction, the key to successfully dealing with damaged soil is to understand soil properties and how to use them to support soil recovery.
A soil primer
What is soil? To the contractor, soil is the foundation upon which we build structures. To the horticulturist, soil is the foundation upon which we build plants. Roy Simonson plowed deeper in the 1957 USDA Yearbook of Agriculture: “Be it deep or shallow, red or black, sand or clay, the soil is the link between the rock core of the earth and the living things on its surface. It is the foothold for the plants we grow. Therein lies the main reason for our interest in soils.”
Where does soil come from? Soil formation is an ongoing, dynamic process that is affected over time by climate, topography, bedrock/parent material, and macro- and microorganisms. The operative word here is “dynamic,” as soil is ever-changing. This is an important point relative to our ability to make corrections to soil that has been damaged by construction activity. Carol Williams, author of Bringing a Garden to Life, said, “Most of all one discovers that the soil does not stay the same, but, like anything alive, is always changing and telling its own story. Soil is the substance of transformation.”
Soil forms naturally from the top-down and from bottom-up. This is why soil looks like a layer cake when viewed in profile. The different layers are called “horizons.” Figure 2 shows the five basic soil horizons: organic (“O” horizon); topsoil (“A” horizon); subsoil (“B” horizon); parent material (“C” horizon); and bedrock (“D” horizon). Organic matter is constantly moving downward in the soil profile to affect horizons beneath the organic horizon. This is particularly important to the development of viable topsoil.
Conversely, although it would seem to defy gravity, material found in the deep horizons is constantly moving upward in the soil profile. Bedrock is continuously fracturing to become parent material, and parent material is always weathering to become subsoil. Ultimately, the subsoil contributes to the topsoil horizon (see sidebar, opposite).
As critical is topsoil is for a healthy landscape, it is also important for landscapers to have a clear understanding of the horizons lying beneath the site. For example, limestone and calcite share chemical names (calcium carbonate) and chemical formulas (CaCO3), and both will eventually cause the pH of topsoil to rise. If the bedrock and parent material horizons are limestone or calcite, the topsoil overlaying these horizons will naturally become alkaline over time. Growing acid-loving plants would require periodical acidification of the soil. Of course, a better solution would be to select plants that are tolerant of alkaline conditions.
What is topsoil?
There is no official, regulated definition for topsoil; it is the soil on top. Thus, it is very important for landscapers to know something about local sources of topsoil. A topsoil harvested a short distance from the installation site is likely to have the same characteristics as the topsoil found on the site. This is not a problem if the local topsoil has desirable characteristics and is being used to replace topsoil lost during construction. However, if the goal is to improve the soil by purchasing and spreading additional topsoil, the quality of the purchased topsoil becomes an important consideration. Landscapers should ask the topsoil supplier to provide the results of a soil test conducted on their product by a reputable soil-testing lab. This is the only credible way to learn the soil’s “pedigree,” including the soil’s pH, cationic exchange capacity (CEC), nutrient condition and texture. We will talk about the importance of learning these soil attributes in this article.
Soil damaged by construction presents a challenge to every landscape professional.
JOE BOGGS
There are three soil properties that are used to describe the general characteristics of soil. These are:
- Physical properties: This includes the way soil is deposited in layers (horizons) as well as the way the soil “feels” (texture) and how it is constructed (consistency and structure). Certain soil problems, such as poor drainage and compaction, are described based on physical properties.
- Chemical properties: Soil chemistry drives many of the nutrient characteristics of soil that are important to plant health, such as pH and cation exchange capacity (CEC).
- Biological properties: The soil is home to a menagerie of micro- and macroorganisms that greatly influence soil structure, a physical property.
It is important to keep in mind that these soil properties do not act independently of one another; they interact to influence the ability of soils to support healthy plants. For example, soils that are high in clay (a physical property) have a greater ability to hold certain nutrients (a chemical property). Conversely, a high percentage of clay reduces soil drainage, which influences the types of organisms (a biological property) that can live in the soil.
Chemistry ABCs
An atom is the smallest form of an element that has all of the chemical properties of that element. (Hydrogen is the simplest element.) Note that an atom is composed of two basic structures: a positively charged nucleus at the center of the atom and negatively charged electrons orbiting the nucleus. With hydrogen, the positive charge of the nucleus is balanced by a single, negatively charged electron.
What happens when an atom has too few or too many electrons? The atom will have either a positive charge (+) or a negative charge (-), and it is called an ion. If an atom has too few electrons to balance the positive charge of the nucleus, the entire atom becomes positively charged (+) and is known as a cation. If the atom has too many electrons, the atom becomes negatively charged (-) and is called an anion. What happens when a cation meets an anion? They act just like opposite ends of bar magnets; they are attracted to one another.
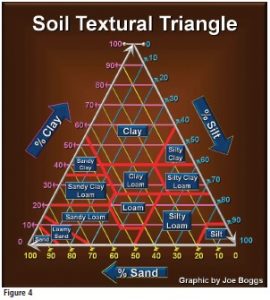
Soil texture
Soil texture is one of the “physical properties” of soil and is based on the relative percentages of the three mineral components of the soil: sand, silt and clay. Texture is literally the way the soil “feels” when rubbed between your fingers. Whether a soil particle is classified as sand, silt or clay depends on the size of the particle. Soil testing labs use a series of screen sieves to separate the different sized mineral particles found in a soil sample. The following particle classification is based on the USDA System:
- Sand: 2.00 to 0.05 mm; the largest particles, which feel “gritty”.
- Silt: 0.05 to 0.002 mm; medium sized particles, which feel soft, silky or “floury”.
- Clay: less than 0.002 mm; the smallest sized particles, which feel “sticky” when wet.
The “Soil Textural Triangle” (Figure 4, left) is used to assign a soil textural classification (textural types) to a soil sample. Once the relative percentages of sand, silt and clay are known for a soil sample, the triangle is used to find where the soil “fits” among the textural types. For example, a soil that has 40 percent sand, 40 percent silt and 20 percent clay would be classified as a “loam.” Loam is generally considered to be the best soil texture for plant growth, while clay is often maligned as the least desirable mineral component of the soil. However, note on the Textural Triangle that loam soil may contain as much as 26.9 percent clay! This illustrates that the best soils have a helping of all three mineral components. It is also important to remember that the only way to change soil texture is to change the relative percentages of the three mineral components of the soil.
What about gypsum and clay soil? Gypsum is calcium sulfate (CaSO4), and it affects the soil by acting on chemical properties, not by changing the physical properties of soil. The idea that gypsum can improve clay soils is based on a misunderstanding. Soils that contain high concentrations of salt (sodic soils) behave similar to soils that contain a high percentage of clay; they both become sticky when wet. Gypsum can improve sodic soils by inducing chemical reactions that release mineral salts from the soil particle; however, it does not change the actual percentage of clay in the soil. On the other hand, gypsum is an excellent source of calcium and, unlike lime-based calcium sources such as dolomitic lime [CaMg(CO3)2], gypsum will not affect soil pH.
Figure 5 (page 23) displays a graph that is frequently used in soil manuals to illustrate the relationship between soil texture and soil moisture. The amount of water in the soil is plotted on the x-axis of the graph, while soil particle size (texture) is plotted on the y-axis. Following are the definitions of the terms used on the graph:
- Gravitation Water: the water that will drain from the soil by gravity. Imagine holding soil that is saturated with water up in the air. Gravitational water is all of the water that will eventually drain by gravity from the soil.
- Field Capacity: the water that remains in the soil after it has been drained by gravity.
- Water Available to Plants: the water that is held in the soil, but is able to be transferred from the soil into the roots of plants.
- Permanent Wilting Point: the water in the soil that is so tightly bound on the surface of soil particles that it is not available to plants.
- Unavailable Water: the soil is practically devoid of all water.
At first glance, the information presented on the graph appears straightforward. After all, the fact that sand drains faster than clay is common sense. However, this is not the most important soil feature that is depicted on the graph. Remember that it is only “Gravitation Water” that drains from the soil. The “Water Available to Plants” refers to water that is being held in the soil against gravity. What is keeping the water from draining from the soil, and why does clay hold on to more water compared to sand and silt?
Demystifying pH
What is pH and why is it important to growing healthy plants? The term “pH” stands for “potential hydrogen.” Water molecules occasionally break apart to release one hydrogen atom (H+), which is a cation, and one hydroxyl molecule (OH-), which is an anion. This is called the “self-ionization of water.” The pH scale, as illustrated in Figure 8, is a measure of the number of H+ ions in the soil. Note that the higher the number of H+ ions, the higher the acidity of the soil. As the number of number of H+ ions in the soil decreases, the soil becomes less acid; it becomes more alkaline (also known as “basic”).
Figure 9 illustrates why knowing the soil’s pH is important to the success of growing healthy plants. The graph shows that soil pH drives soil chemistry. The width of the bands in the graph depicts the availability of nutrients to plants relative to soil pH. The wider the bands, the more available the nutrients are to plants; the narrower the band the less available the nutrients are to plants. Where the bands are narrow, the nutrients may actually be in the soil in concentrations sufficient to support health plants; however, the H+ or OH- ions are “locking up” the nutrients by causing them to bond with other chemicals in the soil to create water insoluble molecules. Thus, the nutrients cannot be carried by water into plant roots.
The self-ionization of water doesn’t happen very often; however, it could be argued that it is the most important chemical reaction that occurs in nature because without it, there would be little to no chemical activity in the soil.
Part of the answer is related to particle size. Water is held on the surface of soil particles; it does not penetrate individual sand, silt or clay particles. Smaller particles have a greater surface-to-volume ratio compared to larger particles. This means that a collection of small particles (clay) will have a greater total surface area to hold on to water compared to a collection of large particles (sand). To imagine how this works, consider how we might increase the surface-to-volume ratio of a table. By cutting the table into pieces, we create smaller table “particles,” and we’ve exposed a larger surface area compared to the original table. The un-cut table would represent sand particles while the small pieces of the cut-up table would represent clay particles.
The second reason clay holds onto more water compared to sand and silt is more subtle. It is related to chemical characteristics of water coupled with certain physical characteristics of clay. For a primer on the basic principles of chemistry, see Chemestry ABCs, page 23.
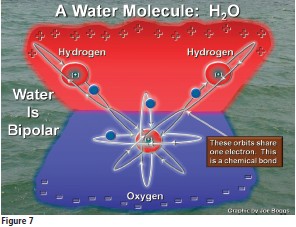
The strange properties of water
Fig. 7 shows a water molecule. The molecule consists of an oxygen atom (O) that is attached to two hydrogen atoms (H2), so the chemical formula for the water is H2O. Oxygen atoms usually exist in nature as anions, so they have a negative electrical charge. Hydrogen atoms usually exist as cations, so they have a positive electrical charge. The H and O atoms were attracted to one another because they have opposite charges; however, note in Fig. 7 that the molecule is held together by the H and O atoms sharing electrons. This is called a chemical bond and it is much stronger than an electrostatic bond, the kind of “bond” that can be illustrated by joining opposite ends of bar magnets.
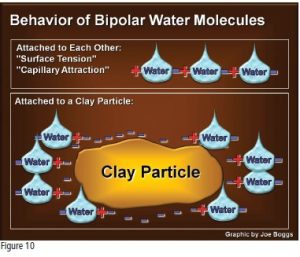
Cationic exchangecapacity (CEC)
The negative charges on the surface of clay particles also play an important role in holding nutrients in the soil. Each clay particle has a specific number of negatively charged sites, and the number of negatively charged sites is constant. Many of the nutrients that are important to plant health are cations; they are positively charged. The cations are attracted to the clay particles and become attached to the negative sites by electrostatic bonds. Remember that an electrostatic bond is not the same as a chemical bond. The clay particles do not share electrons with the cations. It’s just like the weak attachment between oppositely charged ends of bar magnets. The bar magnets can easily be pulled apart; they do not become welded together. Thus, the cations that are attached to clay particles can detach and become available to plants.
The CEC is a measure of the total number of negative sites on all the clay particles in the soil. To visualize this, picture a bus; the seats on the bus represent the negatively charged sites on the clay particle. The passengers on the “CEC bus” are cations. Of course, CEC does not measure the number of seats on a single CEC bus. It measures the total number of electrostatic “seats” on an entire fleet of buses; on all the clay particles in the soil.
What does CEC mean to you? The higher the CEC, the higher the soil’s capacity to hold on to nutrients; the nutrients are not easily washed through the soil by water. Also, for “mineral soils” (those with sand, silt and clay), the higher the CEC, the higher the clay content of the soil. Conversely, a low CEC number means nutrients can be easily washed from the soil by water. This is why potting soils (for example, “soil-less media”) that contain no clay will not retain nutrients. The nutrients must be continually added in order to grow healthy plants. The answer to the age-old question, “Is clay bad for the soil?” is a resounding “No!” Clay acts like a nutrient sponge to hold nutrients until they are needed by plants.
The entire water molecule has a very strange electrostatic property based on the inherent electrical charges of the hydrogen and oxygen atoms. The two hydrogen atoms impart a weak positive charge to the hydrogen side of the molecule, while the oxygen atom imparts a weak negative charge to the oxygen side of the molecule. This causes the water molecule to be bipolar.
The bipolar nature of water molecules means the molecules behave like tiny bar magnets, each with a positive end and a negative end. Water molecules can attach to one another, just like bar magnets. This electrostatic bond is a very weak type of attachment; however, this strange nature of water explains surface tension and is illustrated by water droplets beading up on a flat surface. It is also illustrated by capillary attraction, which is important to the transport of water up the vascular systems of plants (see Fig. 10, page 25).
The strange properties of clay
Clay particles have distinct sites on their surfaces that are negatively charged. What does this mean to the relationship between clay and water? The positive ends of the bipolar water molecules attach to the negative sites on the surface of the clay particles. Thus, clay holds on to more water compared to sand and silt because of electrostatic forces (Fig. 10) and because clay has a larger surface-to-volume ratio.
What does the relationship between soil texture and moisture holding capacity mean to landscape installations? The differences in the way the small clay particles and the much larger silt and sand particles hold on to water means that water movement is disrupted when soils with drastically different particle sizes are placed next to one another. The problem is called “soil incompatibility,” and the outcome can have dramatic consequences on plant cultivation. For example, trying to improve the drainage of clay soils by adding topsoil with a high sand content means that water will not easily flow from the sand into the clay; a perched water table is created.
Now you’re armed with the nuts and bolts. Part II in the July issue will address how to put it all together to help you correct urban soil problems.
Joe Boggs is an assistant professor with Ohio State University Extension, and commercial horticulture educator with OSU Extension, Hamilton County. He can be reached at [email protected]
Jim Chatfield is an associate professor and extension specialist for Ohio State University extension in the department of horticulture and crop science and the Department of plant pathology. He can be reached at [email protected].